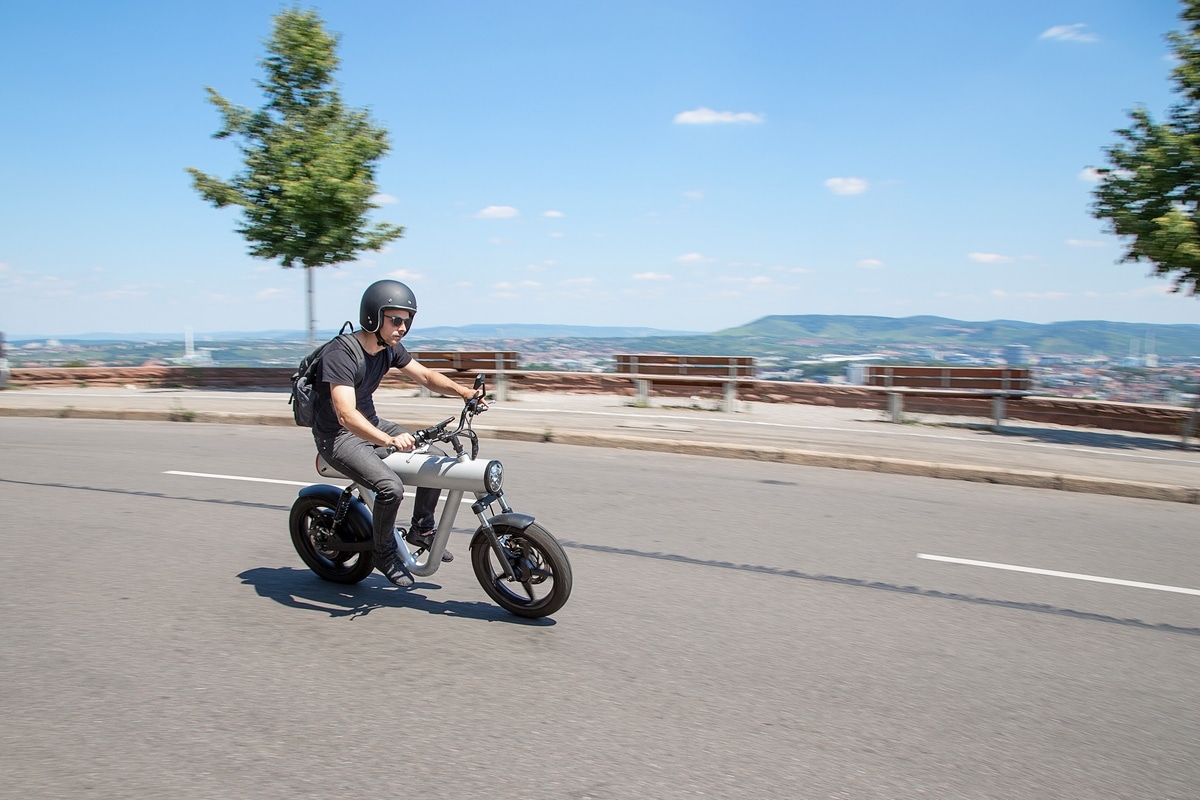
Doubling the distance with a fuel cell range extender
An electric moped with a 150-kilometer (90-mile) range that refuels in under a minute? With a fuel cell and hydrogen tank acting as a range extender, it is feasibly possible. Confirmation of this can be found in a joint study entitled “Pocket Rocket H2” that has been undertaken by DHBW university and SOL Motors in Böblingen, Germany.
Electric bikes and e-scooters have already become part of the urban landscape. Now mopeds are edging into electric propulsion. In fall this year, the eye-catching Pocket Rocket from startup SOL Motors is being launched on the market.
The battery electric model is available in two versions with maximum speeds of 45 km/h (28 mph) or 80 km/h (50 mph). In both cases, the range is between 50 and 80 kilometers (30 and 50 miles) and it takes around three hours for the battery to recharge from a domestic socket. This is usually entirely sufficient for riding the Pocket Rocket on the daily commute to work.
Nevertheless, there are also circumstances where a rider will want to recharge rapidly and travel further. For example, the use of mopeds can be envisaged in disaster response situations where a greater range will be needed along with continuous availability. Conditions that can be met by a fuel cell vehicle.
Electric vehicles: battery or fuel cell?
At the moment, the vast majority of electric vehicles around the world, from e-scooters to light commercial vehicles, are powered by batteries. A fuel cell only comes into play if higher power outputs and large amounts of energy are demanded. Typical examples are heavy-duty vehicles, trains, ships or airplanes. Because the hydrogen tank and the fuel cell are separate, this means that the amount of energy and the power output are decoupled in a fuel cell power system. This gives a higher degree of freedom in terms of the system layout, including for smaller vehicles.
A fuel cell power system cannot dispense with a battery completely since it is needed for starting the system and for regeneration. There are various ways to configure the battery in combination with the fuel cell: If the entire driving power is provided by the battery, the fuel cell acts only as a range extender. Almost the reverse of this would be the option whereby propulsion would be provided purely by the fuel cell and a small starter battery would be used that can temporarily store the braking energy. If both power sources work together, this is called hybrid operation.
Consequently, the Pocket Rocket H2 project focused initially on the issue of configuration since comparable vehicles are not (yet) on the market. The starting point for calculations was the test cycle defined in the Worldwide Harmonised Light Vehicle Test Procedure or WLTP which, together with the vehicle data for the Pocket Rocket (version with maximum 45 km/h), supplies the power and energy from fig. 2. As a result, the decision was made to opt for a fuel cell as a range extender.
Fig. 2: Calculated power and energy requirement of the Pocket Rocket (version with max. 45 km/h) from the WLTP test cycle
Benefits of a fuel cell range extender
When a fuel cell is used as a range extender, its purpose is merely to recharge the battery. Therefore very little has to be done to the battery electric vehicle’s control system. In its capacity as a range extender, the fuel cell only has to supply a power output of up to 1,000 watts; peak loads are covered by the battery. The range, meanwhile, is limited only by the size of the hydrogen tank. For fuel cells in the performance class up to 1,000 watts, simple air cooling is sufficient; from around 2.5 kilowatts upward, a more complex water cooling system is needed. As a range extender, the fuel cell can be operated at constant power while also protecting the battery from exhaustive discharge. Both factors increase the life of these components.
The only disadvantage of the chosen configuration is that the battery has to be large enough to ensure several kilometers can be covered with a power output over 1,000 watts, e.g. for mountain driving.
Demonstrator in the lab
As part of the project, the system comprising the battery and fuel cell range extender was set up as a lab prototype. For this purpose, a proton exchange membrane or PEM fuel cell system from Hydrogen Air Technologies was used (see fig. 3).
Fig. 3: Compact fuel cell system with a 1,000-watt continuous output. The fans for air cooling can be seen in the right of the picture. The hose between the fans is used for nitrogen purging.
The system with its 65 cells is air-cooled by means of simple, speed-controlled fans and supplies the described maximum electrical output of 1,000 watts. The voltage varies, depending on the output level, between 65 volts (idling) and 35 volts (maximum power). The setup is known as a dead-end system, in other words only the amount of hydrogen that will be consumed is supplied.
In the dead-end system, nitrogen accumulates relatively quickly on the hydrogen side (anode) due to diffusion. This nitrogen must then be released via a purging valve. Purging lowers the efficiency of the system as it also carries away unused hydrogen. The fuel cell system under investigation has an efficiency of around 35 percent at 1,000 watts. Converted into hydrogen consumption, this is equivalent to 85 grams of hydrogen an hour.
Electrical connection
The use of the fuel cell system as a range extender results in an extremely simple electrical connection. As illustrated in fig. 4, a DC-DC converter has to adjust the output voltage of the fuel cell to the end-of-charge voltage of the battery. The battery can then be continuously charged at a constant voltage. The fuel cell controller adjusts its output power to the prevailing charging current. The powertrain control unit remains unaffected by the charging carried out by the fuel cell.
Fig. 4: Connection of electrical components of the fuel cell when used as a range extender
Thanks to the fuel cell system, the battery can be reduced from 2.5 kilowatt-hours to 0.35 kilowatt-hours while retaining the same motor output. In principle, the range can then only be limited by the tank volume, in other words the quantity of hydrogen in the tank. The power requirement ascertained by the WLTP test cycle produces, together with the system efficiency, a hydrogen consumption of approximately 200 grams for 100 kilometers (60 miles). This means that the fuel cell version of the Pocket Rocket could travel a distance of 500 kilometers (300 miles) on 1 kilogram of hydrogen!
Concern about pressurized hydrogen tanks
Unfortunately, the storage of hydrogen for motive applications is still unsatisfactory. Hydrogen is around 14 times lighter than air. It therefore has to be compressed in order to store the gas in significant quantities. But even at a pressure of 700 bar, 1 kilogram of hydrogen takes up a volume of almost 40 liters. In addition, a pressurized tank of 700 bar, which stores 1 kilogram of hydrogen, weighs about 24 kilograms. This makes it even more remarkable that the Pocket Rocket H2 is only around 2 kilograms heavier than the battery electric vehicle – and has twice the range.
By reducing the battery from 2.5 kilowatt-hours to 0.35 kilowatt-hours, its weight decreases from roughly 14 kilograms to just around 2 kilograms. In total this works out at around 16 kilograms which is spread across the fuel cell (4 kg), tank (9 kg), battery (2 kg) and other components (1 kg) such as the DC-DC chopper and connectors. The pressurized hydrogen tank is not only the largest component; it is also the heaviest. This is principally due to the high safety requirements for use in road transportation.
These days, high-pressure tanks for hydrogen consist of a polymer liner wrapped in carbon fibers that have been impregnated with epoxy resin. The carbon-fiber layer is several centimeters thick to ensure the desired requirements are met, for instance a burst pressure of 2.35 times the working pressure. Thus only round or cylindrical tanks can be produced for manufacturing reasons. To house the tank on the frame of the Pocket Rocket, more flexible tank geometries would be desirable, though these would exceed all budgets at this point in time.
In the final part of the project, options for housing the components of the range extender on the frame of the Pocket Rocket were explored in a CAD model (see fig. 5).
Fig. 5: Research into the arrangement of individual components of the fuel cell range extender on the frame of the Pocket Rocket H2. The pressurized hydrogen tanks take up the most space.
The battery, which in the battery electric model is located in the upper cross tube, is now much smaller and could migrate into one of the V tubes. In this version, hydrogen would be stored in two tanks, both in the cross tube and in a separate tank. Admittedly, the upper tank on its own would be capable of storing almost all the 350 grams of hydrogen needed to double the range. The second tank would only be used if the hydrogen is to be stored at “only” 350 bar. It should also be noted that refueling 6 kilograms of hydrogen takes four minutes for cars. Refilling the Pocket Rocket H2 would take around 14 seconds.
Conclusion and outlook
The Pocket Rocket H2 project was able to demonstrate how the range of a moped can be doubled through using a fuel cell and a hydrogen tank. Instead of long charging times, the “hydrogen moped” can be refueled in an extremely short amount of time. What is surprising is that it is still possible to reduce the overall weight of the fuel cell Pocket Rocket despite the relatively heavy hydrogen tank because a smaller size of battery is used. In the end, only a minimal adjustment was needed to the control system to facilitate the electrical connection in the range extender version. This makes it particularly suitable for “retrofitting” in battery electric vehicles. At the DHBW’s Horb campus, the project findings have already been translated into the design of delivery drones with fuel cell power systems.
In a follow-up project, the lab setup and the Pocket Rocket will be merged into a true hydrogen moped. The Pocket Rocket H2 project received funding as part of the InnovationChallenge 2021 run by the state of Baden-Württemberg’s ministry for science, research and the arts.
ICM’s innovation contest
The InnovationCampus Future Mobility, which is a joint initiative of Karlsruhe Institute of Technology and the University of Stuttgart, is increasing its involvement with industry through the launch of its first InnovationChallenge Mobility and Production. In November 2021, the quick and straightforward funding format for explorative innovation projects brought together the worlds of industry and academia with the aim of jointly solving seven research questions in the fields of mobility and production. The challenges came from innovation-focused companies while the possible solutions were provided by participating universities. The funding, meanwhile, was awarded in the form of small and compact grants by the InnovationCampus. The new funding format is specially designed for small businesses: In the 2021 tendering round, consortiums of businesses and research organizations received more than EUR 900,000 in support.
Author: Prof. Dr. Volker P. Schulz, Kai Tornow, Prof. Wolf Burger, Manuel Messmer